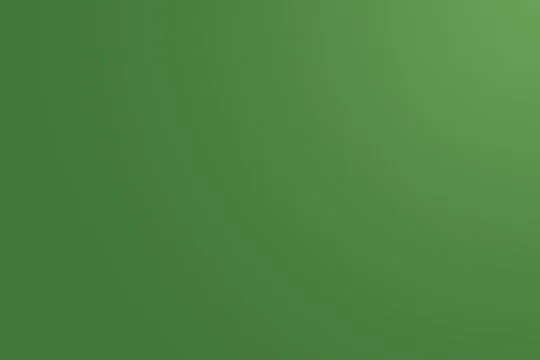
How Does Land Use Affect Soil Chemistry? Impacts of Farming, Urbanisation, and More
Land use shapes soil chemistry in powerful ways. Explore how agriculture, construction, forestry, and grazing alter nutrient levels, pH, and biological health.
The Relationship Between Land Use and Soil Chemistry
Soil chemistry refers to the balance of minerals, nutrients, pH levels, and biological activity within the soil. This chemical makeup is not fixed — it changes constantly, and one of the biggest influences on it is how the land is used. Whether an area is farmed, built on, grazed, or left wild, its use alters the inputs, removals, and biological dynamics that shape the chemical profile of the soil. Understanding how different land uses affect soil chemistry is essential for anyone managing landscapes sustainably or trying to restore degraded ground.
Agricultural Practices and Soil Depletion
In agricultural land, soil chemistry is directly influenced by the removal of nutrients through crop harvesting. When crops are grown repeatedly in the same soil, key nutrients like nitrogen, phosphorus, and potassium are extracted faster than they can be naturally replenished. To compensate, farmers often add fertilisers — both organic and synthetic — which temporarily restore balance but can also cause longer-term shifts. Excess nitrogen can lead to soil acidification over time, while overuse of phosphorus can result in locked-up minerals that plants cannot access.
Tillage also exposes soil to oxygen, speeding up the breakdown of organic matter. This releases nutrients but reduces long-term carbon storage, altering microbial populations and weakening the soil's buffering ability. In monoculture systems, chemical inputs and frequent soil disturbance may lead to imbalances or toxic build-up of specific salts or trace elements.
Grazing and Compaction
Land used for grazing may appear less intensive than arable farming, but it also has a clear impact on soil chemistry. When animals graze, they remove biomass and return nutrients through dung and urine, which can create patches of high nutrient concentration. This uneven nutrient cycling can lead to “hotspots” of nitrogen or potassium that favour aggressive weed species and alter plant diversity.
Heavy grazing also compacts the soil, reducing oxygen levels and disrupting microbial activity. When soil is compacted, water infiltration slows and root penetration is limited, which in turn limits the exchange of gases and the natural cycling of nutrients. The chemical effects of grazing are often tied to the physical changes it brings.
Urbanisation and Soil Contamination
Urban land use tends to degrade soil chemistry rapidly. Construction strips away topsoil, disrupts natural drainage patterns, and introduces pollutants such as heavy metals, hydrocarbons, and alkaline materials from concrete and rubble. These inputs can push pH levels higher, reduce organic content, and disrupt the microbial balance. In many urban environments, soil becomes sealed under pavement or buildings, cutting it off from natural processes like rainfall infiltration and plant growth.
Even green spaces within cities may contain residual contaminants, especially in former industrial areas. Restoring soil in urban zones often requires removal or capping of contaminated layers, followed by the reintroduction of organic matter to rebuild chemical and biological function.
Forestry and Organic Matter Cycles
Forested land behaves differently. In undisturbed forests, leaf litter and decaying wood continuously add organic matter to the soil, building up humus and maintaining a slightly acidic pH. This creates a rich and diverse chemical environment, ideal for fungi and slow-releasing nutrient cycles. However, when forests are cleared or heavily managed, these cycles are broken.
Timber harvesting, especially clear-cutting, removes biomass and disrupts the balance of carbon and nitrogen. If the removed vegetation is not replaced with diverse regrowth, the soil may become depleted or compacted by machinery. In managed plantations, the regular removal of trees and ground cover reduces organic inputs, leading to gradual nutrient loss unless supplemented.
Recreation, Landscaping, and Soil Inputs
Parks, golf courses, and landscaped areas are often treated with fertilisers, herbicides, and irrigation. These inputs change the natural nutrient profile, often leading to higher nitrogen and phosphorus levels than would be found in wild land. Regular mowing and removal of plant material without returning it to the soil can also reduce organic matter over time.
The soil chemistry in these settings is often highly managed but can become unstable if treatments are not balanced or if organic inputs are neglected. This is especially true in compacted lawns or heavily trafficked areas where root growth is limited.
Soil Acidification and Liming Cycles
In agricultural systems, especially those involving heavy nitrogen fertiliser use, repeated applications can lead to soil acidification. Ammonium-based fertilisers and urea convert to nitrate and release hydrogen ions in the process. Over time, this lowers pH, affects cation exchange capacity, and reduces the availability of nutrients like calcium and magnesium. To counteract this, lime is often applied to neutralise acidity — but this creates a chemical feedback loop. Repeated cycles of acidification and liming can destabilise soil chemistry, especially in lighter soils with low buffering capacity.
Without careful monitoring, this loop leads to nutrient imbalances, where some elements become overly concentrated and others become deficient or locked up, even when present in the soil.
Soil Salinity and Irrigated Agriculture
In arid or semi-arid regions, irrigation — particularly flood or poorly drained systems — can lead to salinisation. As water evaporates, salts from the water or fertilisers accumulate near the surface. This increases soil salinity and alters the chemical environment dramatically, affecting osmotic pressure and restricting the ability of plant roots to absorb water and nutrients.
Even in the UK, salinity can be a concern in coastal farming, reclaimed land, or greenhouses using hard water. Changing land use to irrigation-heavy farming can introduce long-term challenges that require deep leaching, drainage redesign, or salt-tolerant crops.
Soil Organic Carbon and Land Cover
One of the most profound chemical impacts of land use change is the shift in soil organic carbon (SOC). Natural land cover, like grassland or forest, maintains steady inputs of organic matter that store carbon in the soil. When these areas are converted to cropland, pasture, or urban areas, that carbon store is often lost. Tillage, reduced root systems, and removal of plant residues speed up decomposition and release carbon as CO₂.
This doesn’t just impact climate — it weakens soil structure, reduces water retention, and affects nutrient holding capacity. Land uses that minimise disturbance, like agroforestry or managed pasture, help stabilise or even increase SOC over time.
Trace Element Dynamics
Changes in land use can also disrupt trace element cycling. For example, long-term grazing without nutrient return can lead to deficiencies in copper or zinc, which are essential for plant health but needed only in small amounts. On the other hand, industrial land use, vehicle emissions, or the use of sewage sludge can introduce excess levels of heavy metals like lead, cadmium, or nickel.
These elements affect microbial life, plant uptake, and can pose toxicity risks to animals and humans if absorbed into crops. Once in the soil, heavy metals persist and are difficult to remove without costly remediation.
Soil Buffering Capacity and Resilience
Soil’s ability to resist chemical change — known as buffering capacity — varies with land use. Natural soils with high organic content and clay tend to resist pH swings, salt accumulation, or nutrient depletion. However, when land is cleared, compacted, or repeatedly fertilised without organic inputs, that buffering capacity is reduced. This makes the soil more reactive and fragile, meaning even small changes in inputs or climate can trigger rapid chemical shifts.
Restoring this resilience involves rebuilding organic matter, improving structure, and managing nutrient inputs more precisely.
Interactions Between Physical and Chemical Changes
Many of the chemical effects of land use are tied closely to physical changes. For example, compacted soil from heavy machinery or grazing reduces air flow, slowing microbial decomposition and shifting nutrient cycles. Erosion strips away topsoil — the layer richest in nutrients and microbial life — and exposes subsoils that may be lower in fertility or higher in toxic elements like aluminium.
Soil chemistry does not change in isolation. It is tightly bound to structure, biology, and water dynamics — all of which are shaped by land use decisions.
Final Word
Land use plays a defining role in shaping soil chemistry. Whether through nutrient removal, compaction, pollution, or changes in organic input, the way we use land directly affects the chemical health and biological balance of the soil. Restoring or preserving soil quality depends on recognising these impacts and adapting practices accordingly. By understanding how soil responds to different uses, it becomes possible to manage it more wisely — for productivity, biodiversity, and long-term sustainability.